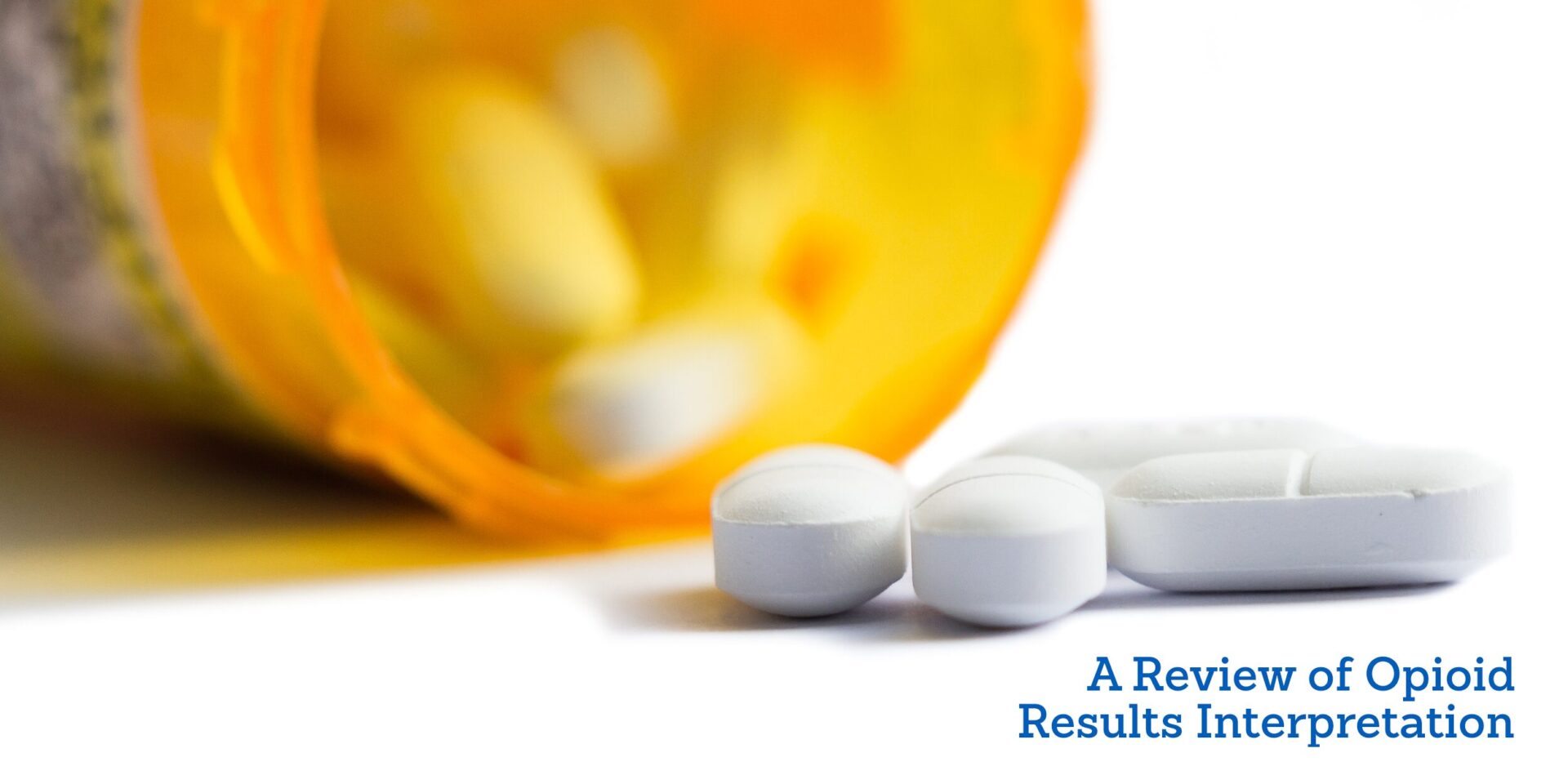
01 Nov What did my Patient Actually Take? An Overview of Opioid Results Interpretation
Opioids refer to all-natural, semi-synthetic, and fully-synthetic drugs that target the opioid receptors to reduce pain. Morphine and codeine are found naturally in opium. The terms “opiates and semi-synthetic opioids” include drugs such as hydrocodone, oxycodone, hydromorphone, and oxymorphone, which are structurally related to codeine and morphine. Synthetic opioids include drugs such as methadone, fentanyl, meperidine, levorphanol, buprenorphine, and tramadol.
Opioids undergo extensive metabolism, both through cytochrome P450 (CYP450) enzymes (phase 1 metabolism) and glucuronidation by uridine diphosphate glucuronosyltransferase (UGT) enzymes (phase 2 metabolism). Pharmacogenetics, drug-drug and drug-food interactions may impact metabolism through these pathways.1-2
Metabolism of Opioids
Many prescription opioids are metabolized to other prescribable opioids (see figure below), and this may complicate the interpretation of test results. Though it would be helpful for interpretation, parent to metabolite ratios do not allow for the identification of the initial opioid ingested, with few exceptions. In some circumstances, metabolites may be present in the absence of parent drug; for example, a patient who ingests codeine could have only detectable morphine in urine.3
Normetabolites of opioids, such as norcodeine, norhydrocodone, and noroxycodone, are unique biomarkers formed after the use of the corresponding drug (i.e., norcodeine after codeine ingestion) and are products of CYP3A4 metabolism.3 Although these metabolites possess weak opioid activity, they are not likely to contribute to the overall analgesic effect.4-6 CYP3A4 metabolism is subject to induction and inhibition by many drug-drug and drug-food interactions, potentially altering opioid normetabolite concentrations.7-11 Drugs that inhibit or induce the CYP3A4 route of metabolism can modify the effect of opioids, leading to either a decrease in analgesic effects or adverse drug effects such as sedation, respiratory depression, and/or death.
Morphine, hydromorphone, and oxymorphone are pharmacologically active and are products of CYP2D6 metabolism of codeine, hydrocodone, and oxycodone, respectively.9-12 Although CYP2D6 cannot be induced, it is subject to inhibition by a host of medications and may also become saturated. CYP2D6 also exhibits a tremendous amount of genetic variability.9,1 The clinical significance of CYP2D6 inhibition is variable and highly dependent on its role in the metabolism of an opioid. CYP2D6 inhibition can lead to an increase in active parent drug or a decrease in active metabolite leading to either a decrease in analgesic effects or adverse drug effects such as sedation, respiratory depression, and/or death.
The drug testing methodology chosen may directly impact patients, as they may be falsely accused of abusing drugs or not being adherent to their prescribed medications.13 While point-of-care testing (POCT) can provide timely presumptive results, there are times when a definitive mass spectrometry analyses (LC-MS/MS or GC-MS) is needed to provide the most appropriate patient care.13
Example of prescribed hydrocodone on an Aegis lab report:
Example of prescribed oxycodone on an Aegis lab report:
Minor Opioid Metabolism
Although patients ingesting a parent drug may excrete only metabolite in urine, there are two notable exceptions:
Metabolism of morphine to hydromorphone (see example below)
Metabolism of codeine to hydrocodone (see example below)
Both metabolic pathways have the potential to result in small proportions of metabolites, which should not exceed parent drug.3 The enzymes responsible for these metabolic pathways have not been identified, and metabolism may not occur in all patients. Patients metabolizing codeine to hydrocodone will typically exhibit hydrocodone concentrations under 5% of the codeine concentration in urine.24 Patients metabolizing morphine to hydromorphone will typically exhibit hydromorphone concentrations under 6% of the morphine concentration in urine.25-30
Metabolism of Synthetic Opioids
Synthetic opioid metabolism also often occurs through the CYP450 enzyme system, with varying responsible enzymes (see table below). Most synthetic opioids have a unique metabolite, typically a normetabolite, which may be detected in biologic specimens.
Metabolite Patterns in Urine
Due to inter-patient variability, there is a broad range of observable patterns of parent drugs and metabolites in urine and oral fluid. Typically, parent drug concentrations exceed metabolites in oral fluid, whereas in urine, the reverse is true. However, this is not always the case, and metabolite ratios should not be broadly used to establish compliance with prescribed drugs or dosages.
Parent Drug Only/No Metabolites Detected
It is possible to observe parent drug presence in urine in the absence of metabolites. The likelihood of such a finding may be increased in patients with impaired metabolism due to genetics or drug-drug/ drug-food interactions. Finding parent-only compounds may suggest recent oral drug ingestion, though concentrations in these cases should typically be low. It is important to note that in excretion studies of single-dose hydrocodone and oxycodone, most subjects’ first urine specimens (0-2 hours after ingestion) had both detectable parent compounds and normetabolites (norhydrocodone and noroxycodone, respectively).31,32
Example of no-metabolites detected comment on the Aegis lab report:
NOTICE: The information above is intended as a resource for health care providers. Providers should use their independent medical judgment based on the clinical needs of the patient when making determinations of who to test, what medications to test, testing frequency, and the type of testing to conduct.
References:
1. Smith HS. Opioid metabolism. Mayo Clin Proc. 2009;84(7):613-24.
2. Smith HS. The metabolism of opioid agents and the clinical impact of their active metabolites. Clin J Pain. 2011;27(9):824-38.
3. Cone EJ, Caplan YH. Urine toxicology testing in chronic pain management. Postgrad Med. 2009;121(4):91-102.
4. Hutchinson MR, Menelaou A, Foster DJ, et al. CYP2D6 and CYP3A4 involvement in the primary oxidative metabolism of hydrocodone by human liver microsomes. Br J Clin Pharmacol. 2004;57:287-97.
5. Lalovic B, Phillips B, Risler LL, et al. Quantitative contribution of CYP2D6 and CYP3A to oxycodone metabolism in human liver and intestinal microsomes. Drug Metab Dispos. 2004;32:447-54.
6. Weinstein SH, Gaylord JC. Determination of oxycodone in plasma and identification of a major metabolite. J Pharm Sci. 1979;68:527–8.
7. Leow KP, Smith MT. The antinociceptive potencies of oxycodone, noroxycodone, and morphine after intracerebroventricular administration to rats. Life Sci. 1994;54:1229-36.
8. Thompson CM, Wojno H, Greiner E, et al. Activation of G-proteins by morphine and codeine congeners: insights to the relevance of O- and N-demethylated metabolites at mu- and delta-opioid receptors. J Pharmacol Exp Ther. 2004;308:547-54.
9. Coller JK, Christrup LL, Somogyi AA. Role of active metabolites in the use of opioids. Eur J Clin Pharmacol. 2009;65:121-39.
10. Armstrong SC, Cozza KL. Pharmacokinetic drug interactions of morphine, codeine, and their derivatives: theory and clinical reality, part II. Psychosomatics. 2003;44:515-20.
11. Caraco Y, Sheller J, Wood AJ. Pharmacogenetic determinants of codeine induction by rifampin: the impact on codeine’s respiratory, psychomotor and miotic effects. J Pharmacol Exp Ther. 1997;281:330-6.
12. Zhou SF, Xue CC, Yu XQ, et al. Clinically important drug interactions potentially involving mechanism-based inhibition of cytochrome P450 3A4 and the role of therapeutic drug monitoring. Ther Drug Monit. 2007;29:687-710.
13. Substance Abuse and Mental Health Services Administration. 2012.
14. Nieminen TH, Hagelberg NM, Saari TI, et al. Rifampin greatly reduces the plasma concentrations of intravenous and oral oxycodone. Anesthesiology 2009;110:1371-8.
15. Lee HK, Lewis LD, Tsongalis GJ, et al. Negative urine opioid screening caused by rifampin-mediated induction of oxycodone hepatic metabolism. Clin Chim Acta. 2006;367:196-200.
16. Otton SV, Schadel M, Cheung SW, et al. CYP2D6 phenotype determines the metabolic conversion of hydrocodone to hydromorphone. Clin Pharmacol Ther. 1993;54:463-72.
17. Otton SV, Wu D, Joffe RT, et al. Inhibition by fluoxetine of cytochrome P450 2D6 activity. Clin Pharmacol Ther. 1993;53:401-9.
18. Somogyi AA, Barratt DT, Coller JK. Pharmacogenetics of opioids. Clin Pharmacol Ther. 2007;81:429-44.
19. Kaplan HL, Busto UE, Baylon GJ, et al. Inhibition of cytochrome P450 2D6 metabolism of hydrocodone to hydromorphone does not importantly affect abuse liability. J Pharmacol Exp Ther. 1997;281:103-8.
20. Crews KR, Gaedigk A, Dunnenberger HM. Clinical pharmacogenetics implementation consortium (CPIC) guidelines for codeine therapy in the context of cytochrome P450 2D6 (CYP2D6) genotype. Clin Pharmacol Ther. 2012;91(2):321-6.
21. Elkader A, Sproule B. Buprenorphine: clinical pharmacokinetics in the treatment of opioid dependence. Clin Pharmacokinet. 2005;44:661-80.
22. Labroo RB, Paine MF, Thummel KE, et al. Fentanyl metabolism by human hepatic and intestinal cytochrome P450 3A4: implications for interindividual variability in disposition, efficacy, and drug interactions. Drug Metab Dispos. 1997;25:1072-80.
23. Ramirez J, Innocenti F, Schuetz EG, et al. CYP2B6, CYP3A4, and CYP2C19 are responsible for the in vitro N-demethylation of meperidine in human liver microsomes. Drug Metab Dispos. 2004;32:930-6.
24. Totah RA, Sheffels P, Roberts T, et al. Role of CYP2B6 in stereoselective human methadone metabolism. Anesthesiology. 2008;108:363-74.
25. Somogyi AA, Menelaou A, Fullston SV. CYP3A4 mediates dextropropoxyphene N-demethylation to nordextropropoxyphene: human in vitro and in vivo studies and lack of CYP2D6 involvement. Xenobiotica. 2004;34:875-87.
26. Wolters Kluwer Health. Facts & Comparisons® eAnswers. St. Louis, MO.
27. Subrahmanyam V, Renwick AB, Walters DG, et al. Identification of cytochrome P-450 isoforms responsible for cis-tramadol metabolism in human liver microsomes. Drug Metab Dispos. 2001;29:1146-55.
28. Klotz U. Tramadol—the impact of its pharmacokinetic and pharmacodynamic properties on the clinical management of pain. Arzneimittelforschung. 2003;53:681-7.
29. Purdue Pharma L.P. Butrans prescribing information. Stamford, CT, June 2010.
30. Oyler JM, Cone EJ, Joseph RE Jr, Huestis MA. Identification of hydrocodone in human urine following controlled codeine administration. J Anal Toxicol. 2000;24:530-5
31. Reisfield GM, Chronister CW, Goldberger BA, Bertholf RL. Unexpected urine drug testing results in a hospice patient on high-dose morphine therapy. Clin Chem. 2009;55(10):1765-9.
32. Hughes MM, Atayee RS, Best BM, et al. Observations on the metabolism of morphine to hydromorphone in pain patients. J Anal Toxicol. 2012;36:250-56.